A group of U.S. scientists have developed a quantitative method for the determination of metal complexes with dissolved organic matter (DOM) in the environment. The method is using an LC-ICP-MS approach with gradient compensation for providing consistent sensitivity over the whole chromatographic separation.
Background:Dissolved organic matter (DOM) play an important role in the biogeochemical cycling of elements by controlling the solubility, reactivity, and bioavailability of trace metals in soil and aquatic systems. Different functional groups within the highly complex DOM bind to a vast array of trace metals, including Fe, Co, Ni, Cu, Zn, Cd, and Pb, thereby stabilizing dissolved metals across a range of concentrations. Unfortunately, the mechanisms that govern the metal-binding properties of DOM remain only poorly understood. In part this is due to the complexity of DOM being the result of decaying detritus and metabolites of microbes, fungi, and plants. Resolving distinct fractions of DOM and measuring the concentrations of metals bound to those fractions remains a major analytical challenge. To answer this challenge calls for quantitative methods that can detect multiple metals bound to compounds within chemically distinct fractions of complex organic mixtures.
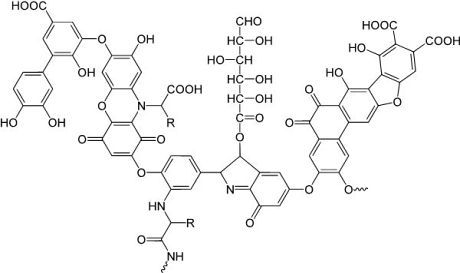
Figure 1:
Model structure of a humic acid (one component of DOM), having a variety of components including quinone, phenol, catechol, and sugar moieties
Reverse phase liquid chromatography (RP-LC) with online inductively coupled plasma mass spectrometry (ICP–MS) has been previously used to quantify specific organic–metal complexes in marine and terrestrial environments. However, its use for quantitative multi-element detection has been limited by variation in ICP–MS elemental response during the chromatographic gradient elution. Furthermore, the effects of chromatographic stationary phase on the separation and recovery of metal-DOM complexes are largely unknown.
The new study:A group of U.S scientists now have significantly advanced the use of a compensation gradient to accurately quantify multiple metals within chemically distinct fractions of DOM. A second HPLC pump delivered a post-column compensation gradient, which oppositely mirrored the separation gradient, resulting in a constant flux of methanol to the ICP–MS. A self-developed software was used for analysing the LC-ICP-MS data, performing internal standard correction, generate calibration curves and calculate concentrations of metals within distinct DOM fractions. They further studied the effects of four stationary phases (C18, amide, HS F5, and phenyl) on the recovery and separation of DOM-bound metals. All stationary phases retained metals to varying extents. On the C18 and amide columns, recoveries of Co, Ni, and Cu were generally higher than recoveries of Zn, Cd, and Pb, and the recovery of Pb was notably low (<60% on C18).
A C18 stationary phase, which turned out to be the best choice for multi-element analysis of metals complexed with DOM was then used to quantitatively determine metal concentrations within distinct fractions of DOM from different soil redox environments. The metal-binding properties of DOM differed substantially between the unsaturated and saturated soil horizons, reflecting differences in DOM composition and metal-binding properties. The authors suggested that these differences indicate that redox conditions may be a major determinant of metal–organic speciation in soils.
The authors concluded that their method provides an analytical foundation for advancing our understanding of trace metal biogeochemistry.
The original publication

Christian Dewey, Daniel I. Kaplan, Scott Fendorf, and Rene M. Boiteau,
Quantitative Separation of Unknown Organic–Metal Complexes by Liquid Chromatography–Inductively Coupled Plasma-Mass Spectrometry, Anal. Chem., 95/20 (2023) 7960-7967.
DOI: 10.1021/acs.analchem.3c00696
Related studies
R.M. Boiteau, D.J. Repeta,
Slow Kinetics of Iron Binding to Marine Ligands in Seawater Measured by Isotope Exchange Liquid Chromatography-Inductively Coupled Plasma Mass Spectrometry. Environ. Sci. Technol., 56 (2022) 3770– 3779,
DOI: 10.1021/acs.est.1c06922
K. Sommer, M. Sperling, U. Karst,
Species-Dependent Interaction of Gd-Based Contrast Agents with Humic Substances. Chemosphere, 300 (2022) 134528,
DOI: 10.1016/j.chemosphere.2022.134528
P. Lin, C. Xu, D.I. Kaplan, H. Chen, C.M. Yeager, W. Xing, L. Sun, K.A. Schwehr, H. Yamazaki, Y. Saito-Kokubu, P.G. Hatcher, P.H. Santschi,
Nagasaki Sediments Reveal That Long-Term Fate of Plutonium Is Controlled by Select Organic Matter Moieties. Sci. Total Environ., 678 (2019) 409– 418,
DOI: 10.1016/j.scitotenv.2019.04.375
R.M. Boiteau, S.J. Fansler, Y. Farris, J.B. Shaw, D.W. Koppenaal, L. Pasa-Tolic, J.K. Jansson,
Siderophore Profiling of Co-Habitating Soil Bacteria by Ultra-High Resolution Mass Spectrometry. Metallomics, 11 (2019) 166– 175,
DOI: 10.1039/C8MT00252E
D.I. Kaplan, C. Xu, S. Huang, Y. Lin, N. Tolić, K.M. Roscioli-Johnson, P.H. Santschi, P.R. Jaffé,
Unique Organic Matter and Microbial Properties in the Rhizosphere of a Wetland Soil. Environ. Sci. Technol., 50 (2016) 4169– 4177,
DOI: 10.1021/acs.est.5b05165
S.M. Kraemer, O.W. Duckworth, J.M. Harrington, W.D.C. Schenkeveld,
Metallophores and Trace Metal Biogeochemistry. Aquat. Geochem., 21 (2015) 159– 195,
DOI: 10.1007/s10498-014-9246-7
C. Xu, S. Zhang, D.I. Kaplan, Y.-F. Ho, K.A. Schwehr, K.A. Roberts, H. Chen, N. DiDonato, M. Athon, P.G. Hatcher, P.H. Santschi,
Evidence for Hydroxamate Siderophores and Other N-Containing Organic Compounds Controlling 239,240 Pu Immobilization and Remobilization in a Wetland Sediment. Environ. Sci. Technol. 49 (2015) 11458– 11467,
DOI: 10.1021/acs.est.5b02310
R.M. Boiteau, J.N. Fitzsimmons, D.J. Repeta, E.A. Boyle,
Detection of Iron Ligands in Seawater and Marine Cyanobacteria Cultures by High-Performance Liquid Chromatography-Inductively Coupled Plasma-Mass Spectrometry. Anal. Chem., 85 (2013) 4357– 4362,
DOI: 10.1021/ac3034568
W. Tan, J. Xiong, Y. Li, M. Wang, L. Weng, L.K. Koopal,
Proton Binding to Soil Humic and Fulvic Acids: Experiments and NICA-Donnan Modeling. Colloids Surf A, 436 (2013) 1152–1158,
DOI: 10.1016/j.colsurfa.2013.08.010
M. Gledhill, K.N. Buck,
The Organic Complexation of Iron in the Marine Environment: A Review. Front Microbiol., 3 (2012) 1– 17,
DOI: 10.3389/fmicb.2012.00069
A.M. Craven, G.R. Aiken, J.N. Ryan,
Copper(II) Binding by Dissolved Organic Matter: Importance of the Copper-to-Dissolved Organic Matter Ratio and Implications for the Biotic Ligand Model. Environ. Sci. Technol., 46 (2012) 9948– 9955,
DOI: 10.1021/es301015p
O.J. Lechtenfeld, B.P. Koch, W. Geibert, K.U. Ludwichowski, G. Kattner,
Inorganics in Organics: Quantification of Organic Phosphorus and Sulfur and Trace Element Speciation in Natural Organic Matter Using HPLC-ICPMS. Anal. Chem., 83 (2011) 8968– 8974,
DOI: 10.1021/ac201765a
E. Tipping,
Cation Binding by Humic Substances; Cambridge University Press, Cambridge 2004
M. Haitzer, G.R. Aiken, J.N. Ryan,
Binding of Mercury(II) to Dissolved Organic Matter: The Role of the Mercury-to-DOM Concentration Ratio. Environ. Sci. Technol., 36 (2002) 3564– 3570,
DOI: 10.1021/es025699i
L.K. Koopal, W.H. van Riemsdijk, D.G. Kinniburgh,
Humic Matter and Contaminants. General Aspects and Modeling Metal Ion Binding. Pure Appl. Chem., 73 (2001) 2005– 2016,
DOI: 10.1351/pac200173122005
J.P. Gustafsson,
Modeling the Acid–Base Properties and Metal Complexation of Humic Substances with the Stockholm Humic Model. J. Colloid Interface Sci., 244 (2001) 102– 112,
DOI: 10.1006/jcis.2001.7871
D.G. Kinniburgh, W.H. van Riemsdijk, L.K. Koopal, M. Borkovec, M.F. Benedetti, M.J. Avena,
Ion Binding to Natural Organic Matter: Competition, Heterogeneity, Stoichiometry and Thermodynamic Consistency. Colloids Surf. A, 151 (1999) 147– 166,
DOI: 10.1016/S0927-7757(98)00637-2
D.L. Sedlak, J.T. Phinney, W.W. Bedsworth,
Strongly Complexed Cu and Ni in Wastewater Effluents and Surface Runoff. Environ. Sci. Technol., 31 (1997) 3010– 3016,
DOI: 10.1021/es970271i
last time modified: September 18,, 2024