Researchers from the University of Ottawa studied the growth of purple bacteria under mercury exposure and found that Hg(II) can fulfil a physiological function in these bacteria by acting as an electron sink to maintain redox homeostasis during photosynthetically energy gain.
Background:The element mercury (Hg) in its different forms (species) is extremely toxic to most organisms, including humans. Its toxic effects are thought to be due to its ability to bind to certain key metabolic enzymes thereby blocking their function. Because of its extreme toxicity, it has long been thought that mercury had no beneficial biological functions in the living world at all. At least that was presumption until a research team published the first
evidence that a unique group of organisms can not only tolerate certain
levels of the toxin, but actually benefit by its presence.
The new study:
In a paper published this month in Nature Geoscience, D.S. Gregoire and A.J. Poulain from the University of Ottawa show that photosynthetic microorganisms called purple non-sulphur bacteria can use mercury as an electron acceptor during photosynthesis. These bacteria gain energy by a primitive form of photosynthesis that differs from the type common to higher plants. In the case of photosynthesis in plants, water is used as an electron donor, with carbon dioxide the electron acceptor. The result of this process is the production of sugars, the release of oxygen, and the removal of carbon dioxide from the air.
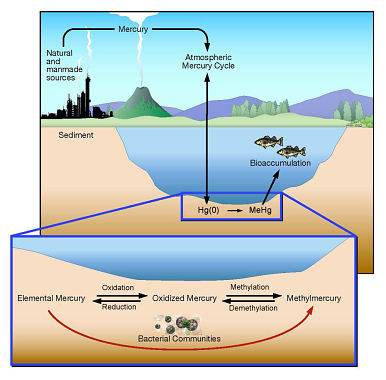
Figure:
Microbial processes that convert elemental mercury into methylmercury.
Credit: Department of Energy's Oak Ridge National Laboratory Purple non-sulphur bacteria, on the other hand, usually live in aqueous anaerobic environments where light is available to them. Instead of water they use hydrogen as the electron donor, and an organic molecule such as glycerol or fatty acids, as the electron acceptor. This also results in the production of sugars, but does not release oxygen or remove carbon dioxide from the atmosphere. This process also generates too many electrons for their organic electron donor to handle, leading to oxidative stress with the potential for damage to other molecules in the cell.
The researcher showed that purple bacteria grow better in the presence of mercury. The reason seems to be that the bacteria use the mercury to accept those extra electrons, reducing the reactive mercury species Hg(II) to elemental mercury Hg°. By this reduction, the availability of mercury for forming methylmercury by biomethylation is reduced, and it partly evaporates away into the atmosphere.
It’s quite possible that the impact of mercury reduction by photosynthesis may extend far beyond the health of these unusual little microbes. Jeffry K. Schaefer, in the Department of Environmental Sciences at Rutgers University speculates that, “By limiting methyl-mercury formation and accumulation in aquatic food webs from microorganisms to fish, this process may even contribute to less toxic mercury ultimately ending up on our dinner plates.”
The new study:

D.S. Gregoire, A.J. Poulain,
A physiological role for HgII during phototrophic growth, Nature Geoscience, 9/2 (2016) 121–125.
DOI: 10.1038/ngeo2629
Related studies:

Haiyan Hu, Hui Lin, Wang Zheng, Stephen J. Tomanicek, Alexander Johs, Xinbin Feng, Dwayne A. Elias, Liyuan Liang, Baohua Gu,
Oxidation and methylation of dissolved elemental mercury by anaerobic bacteria, Nature Geosci., 6 (2013) 751–754.
DOI: 10.1038/ngeo1894 
M.J. Colombo, J. Ha, J.R. Reinfelder, T. Barkay, N. Yee,
Anaerobic oxidation of Hg(0) and methylmercury formation by Desulfovibrio desulfuricans ND132, Geochim. Cosmochim. Acta, 112 (2013) 166–177.
DOI: 10.1016/j.gca.2013.03.001

T. Barkay, K. Kritee, E. Boyd, G. Geesey,
A thermophilic bacterial origin and subsequent constraints by redox, light and salinity on the evolution of the microbial mercuric reductase, Environ. Microbiol., 12 (2010) 2904–2917.
DOI: 10.1111/j.1462-2920.2010.02260.x 
A.D. Anbar,
Elements and evolution, Science, 322 (2008) 1481–1483.
DOI: 10.1126/science.1163100 
W.F. Fitzgerald, C.H. Lamborg, C.R. Hammerschmidt,
Marine biogeochemical cycling of mercury, Chem. Rev., 107 (2007) 641–662.
DOI: 10.1021/cr050353m 
T. Peretyazhko, L. Charlet, B. Muresan, V. Kazimirov, D. Cossa,
Formation of dissolved gaseous mercury in a tropical lake (Petit-Saut Reservoir, French Guiana), Sci. Total Environ., 364 (2006) 260–271.
DOI: 10.1016/j.scitotenv.2005.06.016 
Jeffra K. Schaefer, Jane Yagi, John R. Reinfelder, Tamara Cardona, Kristie M. Ellickson, Shoshana Tel-Or, Tamar Barkay,
Role of the Bacterial Organomercury Lyase (MerB) in Controlling Methylmercury Accumulation in Mercury-Contaminated Natural Waters, Environ. Sci. Technol., 38/16 (2004) 4304–4311.
DOI: 10.1021/es049895w
A.J. Poulain,
M. Amyot, D. Findlay, S. Telor, T. Barkay,
H. Hintelmann,
Biological and photochemical production of dissolved gaseous mercury in a boreal lake, Limnol. Oceanogr., 49 (2004) 2265–2275.
DOI: 10.4319/lo.2004.49.6.2265 
E. Lanzillotta, C. Ceccarini, R. Ferrara, F. Dini, F.F. Frontini, R. Banchetti,
Importance of the biogenic organic matter in photo-formation of dissolved gaseous mercury in a culture of the marine diatom Chaetoceros sp., Sci. Total Environ., 318 (2004) 211–221.
DOI: 10.1016/S0048-9697(03)00400-5
Jeffra K. Schaefer, Jaroslaw Letowski, Tamar Barkay,
'mer -Mediated Resistance and Volatilization of Hg(II) Under Anaerobic Conditions', Geomicrobiol. J., 19/1 (2002) 87—102.
DOI: 10.1080/014904502317246192
L.B. Cleckner, C.C. Gilmour, J.P. Hurley, D.P. Krabbenhoft,
Mercury methylation in periphyton of the Florida Everglades, Limnol. Oceanogr., 44 (1999) 1815–1825.
DOI: 10.4319/lo.1999.44.7.1815

F.M.M. Morel, A.M.L. Kraepiel,
M. Amyot,
The chemical cycle and bioaccumulation of mercury, Annu. Rev. Ecol. Syst., 29 (1998) 543–566.
DOI: 10.1146/annurev.ecolsys.29.1.543
M. Amyot, G. Mierle, D. Lean, D.J. McQueen,
Effect of solar radiation on the formation of dissolved gaseous mercury in temperate lakes, Geochim. Cosmochim. Acta, 61 (1997) 975–987.
DOI: 10.1016/S0016-7037(96)00390-0 
R.P. Mason, J.R. Reinfelder, F.M.M. Morel,
Uptake, toxicity, and trophic transfer of mercury in a coastal diatom, Environ. Sci. Technol., 30 (1996) 1835–1845.
DOI: 10.1021/es950373d 
R.P. Mason, F.M.M. Morel, H.F. Hemond,
The role of microorganisms in elemental mercury formation in natural waters., Wat. Air Soil Pollut., 80, (1995) 775–787.
DOI: 10.1007/BF01189729
D. Ben-Bassat, A.M. Mayer,
Volatilization of mercury by algae, Physiol. Plant., 33 (1975) 128–132.
DOI: 10.1111/j.1399-3054.1975.tb03779.x
Related EVISA Resources
Link Database: Toxicity of Organo-mercury compounds
Link Database: Mercury exposure through the diet
Link Database: Environmental cycling of methylmercury
Link Database: Environmental cycling of inorganic mercury
Link Database: Environmental pollution of methylmercury
Link Database: Environmental pollution of inorganic mercury
Link Database: Toxicity of mercury
Related EVISA News
August 6, 2013: Bacterial methylation of mercury not only starting from oxidized mercury
February 8, 2013: ORNL scientists solve mystery about mercury methylation
June 17, 2012: Factors Affecting Methylmercury Accumulation in the Food Chain
October 9, 2006: Linking atmospheric mercury to methylmercury in fish
February 17, 2006: Study shows link between clear lakes and methylmercury contamination in fish last time modified: September 24, 2024